Thank you for visiting nature.com. You are using a browser version with limited support for CSS. To obtain the best experience, we recommend you use a more up to date browser (or turn off compatibility mode in Internet Explorer). In the meantime, to ensure continued support, we are displaying the site without styles and JavaScript.
Scientific Reports volume 13, Article number: 8140 (2023 ) Cite this article 4,4′-Dimethoxytriphenylmethyl Chloride
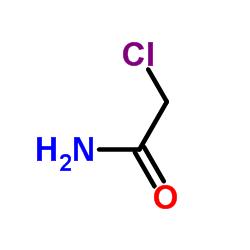
In present work, design and synthesis of a novel pseudo-homogeneous catalyst is described. For this purpose, amine-functionalized graphene oxide quantum dots (N-GOQDs) were prepared from graphene oxide (GO) by a facile one-step oxidative fragmentation approach. The prepared N-GOQDs were then modified with quaternary ammonium hydroxide groups. Various characterization techniques clearly revealed that the quaternary ammonium hydroxide‐functionalized GOQDs (N-GOQDs/OH−) have been successfully synthesized. TEM image revealed that the GOQDs particles are almost regularly spherical in shape and mono-dispersed with particle sizes < 10 nm. The efficiency of the synthesized N-GOQDs/OH− as a pseudo-homogeneous catalyst in epoxidation of α,β-unsaturated ketones in the presence of aqueous H2O2 as an oxidant at room temperature was investigated. The corresponding epoxide products were obtained in good to high yields. This procedure has the advantages of a green oxidant, high yields, involvement of non-toxic reagents and reusability of the catalyst without discernible loss in activity.
Sarieh Momeni & Ramin Ghorbani‑Vaghei
Mujeeb Khan, Mohammed Rafi Shaik, …Muhammad Nawaz Tahir
Virgil D. Lower Jr., Cybele Riesse L. Santos, ... Chun-Hu Chen
In an era of new technologies, a safe and healthy living environment has become of great importance; as a result, the availability of more environmentally friendly chemical approaches for organic compounds production would also constitute an added advantage. The last two centuries have witnessed significant advances in the development of cost effective catalysts for organic transformation focusing on the some aspects of green chemistry1,2.
A pseudo-homogeneous catalyst has been considered as a catalytic system that the catalyst surface is exactly exposed to the substrates i.e. that there is no obvious phase differentiation between substrates and the catalyst and performs like a homogeneous catalyst. However, compared with homogeneous catalysts, the pseudo-homogeneous catalyst could be easily separated from reaction medium and recovered as is characteristic in heterogeneous catalysis. In turn, performance and usability of the catalytic system would be improved since the pseudo-homogeneous system will have the advantages of both the homogeneous and heterogeneous catalysis3,4.
Graphene oxide quantum dots (GOQDs), an emerging category of zero-dimensional nanomaterials, are defined as those oxygen-rich carbonaceous layered materials with constituent dimensions less than 20 nm5. GOQDs have shown promising potentials in electrochemical sensing, photocatalysis, bioimaging, biosensing, light-emitting diodes, and catalysis due to their exceptional properties such as remarkable luminescence, existence cost-effective methods to produce, easy functionalizing ability, very good solubility and stability in water, low toxicity and good biocompatibility6,7,8,9. It is interesting to note that GOQDs can be produced efficiently from various commercially available carbon sources including graphite powder, graphene oxide sheets, carbon fibers, citric acid, plant materials, such as mango leaves and so forth6.
Use of GOQDs as a support for active sites can be surprising since these catalytic systems allow the catalytic reaction occur under pseudo‐homogeneous conditions. Therefore, the catalytically active species can be suspended indefinitely due to the small particle size of CQDs and designed functional groups on it, and catalyst and reactants are in a same phase, so the system can work similarly to a homogeneous catalyst with the added advantage of being recoverable effortlessly by dialysis membrane. Furthermore, the thin sheet of GOQDs contain a range of reactive oxygen functional groups on their surface, which provides high (aqueous) solubility and considerable potential for facile modification10,11,12. On the whole, surface modification techniques can offer exciting possibilities for change the surface of GOQDs for particular applications13. Recently, GOQDs supported catalysts have been explored in organic transformations and they demonstrated excellent results. Rezaei et al. were able to perform the selective oxidative cracking of alkenes to aldehydes using the carbon quantum dots supported ionic liquids14. Immobilized tungstate ions on the surface of carbon quantum dots have been successfully applied in oxidative scission of alkenes and selective oxidation of alcohols to corresponding aldehydes15,16,17. Pd and Ag nanoparticles have also stabilized on carbon quantum dots and the prepared catalyst was utilized as an efficient catalyst for promoting the Suzuki–Miyaura coupling reaction18.
Epoxides have emerged as an extremely useful class of organic compounds with high synthetic utility. In organic chemistry, epoxides are useful and versatile synthon so that can be converted into a wide variety of valuable compounds with good pharmaceutical profiles19. Epoxides compounds are also a broad family of monomers for production of various types of polymers. A number of epoxides derivatives have been reported to show anticancer, antibiotic and protease inhibition activities20,21,22,23. Therefore, academic researchers have been giving much concentration to preparation epoxides-containing compounds.
Epoxidation of carbon–carbon double bonds is one of the most fundamental reactions. This transformation has a wide scope of applications in synthetic organic chemistry24,25. Despite the large number of undesirable environmental and economic effects continue to use stoichiometric oxidations such as sodium peroxide, sodium hypochlorite, m-chloroperbenzoicacid, oxone, sodium chlorite, sodium perborate tetrahydrate, dimethyldioxirane, cyclohexylidenebishydroperoxide and trichloroisocyanuric acid26,27,28,29,30,31,32,33,34. During the recent decade, catalytic protocols based on aqueous hydrogen peroxide (H2O2), air and pure oxygen as environmentally friendly, low-cost and readily available oxidants, are being considered35. Epoxidation of α,β-unsaturated ketones occurs in the presence of hydrogen peroxide under basic conditions and produces epoxy carbonyl compounds36. Representative examples of such systems are ([C4MIm][PF6]) ionic liquid/H2O237, [Al(H2O)6]3+/H2O238, (CTP)3VMo12O40/H2O239, tetrabutylammonium peroxydisulfate/H2O240, [L-Aaemim]Br ionic liquid/H2O241, poly(L-Leucine)/H2O242 and primary amine salts/H2O243.
Although, great strides have been made in the development of affordable catalysts for the epoxidation of α,β-unsaturated ketones approach focusing on the principles of green chemistry, however, it continues to be one of the most interesting areas in scientific literature.
As part of our continuing investigations to develop green catalysts for organic transformations and with the aim of further demonstrating the catalytic potential of GOQDs, on the first step of this study, N-GOQDs were prepared from graphene oxide by an oxidative fragmentation approach. Subsequently, the prepared N-GOQDs modified with quaternary ammonium hydroxide groups. The catalytic activity of this pseudo-homogeneous catalyst (N-GOQDs/OH-) was evaluated in the epoxidation of α,β-unsaturated ketones in the presence of aqueous H2O2 as an oxidant at room temperature.
Used solvents and chemicals were supplied from Fluka (Switzerland) or Merck (Germany), and used without any further purification. Deionized (DI) water was applied in all tests. The Fourier-Transform Infrared Spectroscopy (FT-IR) spectra of the samples were recorded with the KBr pellet method by PerkinElmer PE-1600-FTIR spectrometer. Transmission electron microscopy (TEM) was investigated on an EM 208S (PHILIPS) 100 kV microscope with tungsten filament and Zeiss‐EM10C (Germany) operating at 100 kV with samples on formvar carbon coated grid Cu mesh 300. The prepared samples were investigated by field emission scanning electron microscope (FESEM) (FESEM TESCAN MIRA 3, Czech). The 1H NMR analysis was carried out with a BRUKER DRX-250 AVANCE spectrometer at 250.0 MHz. The optical characteristics of samples were measured by Shimadzu UV 2100 151PC UV–visible spectrophotometer at room temperature. Energy-dispersive X-ray spectroscopy (EDX) analysis were carried out on a SIGMA VP 500 (Zeiss) microscope equipped with an EDX measurement system.
First, the graphene oxide sheets have been prepared by the predominant modified Hummer’s method. Briefly, concentrated H2SO4 (15 mL) was added to a mixture of graphite (0.3 g) and NaNO3 (0.3 g), and the mixture was cooled to 0 °C in an ice‐salt bath. Under stirring, KMnO4 (1.5 g) was added slowly to the suspension over 2 h at 0–10 °C with ice‐salt bath cooling. The mixture was warmed to 35 °C and stirred for 30 min, and the resulting solution was diluted by slowly adding 30 mL of water under stirring. Then the reaction was stirred under reflux for 15 min at 98 °C. After cooling to room temperature, the resulting mixture was treated with 30% H2O2 solution (7 mL). The mixture was washed with HCl and H2O respectively, followed by centrifugation and drying, graphene oxide was thus obtained (0.35 g)44.
0.02 g of as-prepared GO was dispersed in water (5 mL) under sonication conditions. N-(aminomethyl)methanediamine (1 mL) and H2O2 (40 mL, 30% solution in H2O). The mixture was then transferred into a single-neck, flat-bottom round flask and heated at 80 °C for 8 h. The product was then cooled to room temperature and was centrifuged to remove the largest particles and untreated GO sheets. Ultimately, the dark yellow solution was dialyzed using a dialysis membrane (100 Da) to remove the unreacted starting materials, then concentrated under vacuum, giving final volume of 5 mL45.
5 mL methanol and 0.2 mL methyl iodide were added to 5 mL of N-GOQDs solution was. The mixture was stirred under reflux at 25 °C for 24 h. The product was then dialyzed using a dialysis membrane (100 Da) to remove the unreacted methyl iodide. Ultimately, 5 mL of quaternary ammonium iodide‐functionalized N-GOQDs (N-GOQDs/I) was treated with 3 mL KOH (0.1 M) at room temperature for 2 h to obtain quaternary ammonium hydroxide‐functionalized GOQDs (N-GOQDs/OH−)46. Finally, the resulting solution was dialyzed using a dialysis membrane (100 Da) to remove any excess KOH, then was concentrated under vacuum to a final volume of 5 mL.
The catalysis experiments for the N-GOQDs/OH− was carried out in a 1,4-dioxane:water (1:1) mixture, using 1:3 α,β-unsaturated ketones:H2O2 mole ratio. The α,β-unsaturated ketone (1 mmol) was introduced into a round‐bottom flask equipped, to which N-GOQDs/OH− (4 mL of aqueous solution) and the mixture solvent (5 mL) were subsequently added. Then, aqueous 30% H2O2 solution (3 mmol) was added, the reaction solution was stirred (at 1000 rpm) at ambient temperature for 24 h. After detecting the completion of the reaction using thin-layer chromatography (TLC) (n-hexane/ethyl acetate, 2/1), the reaction mixture was diluted with water (5 mL), and the product was extracted with ethyl acetate (3 × 5 mL). The extracted catalyst in the aqueous layer was concentrated under vacuum to a final volume of 4 mL to reuse in another run. On the other hand, the organic phase (ethyl acetate layer) containing unreacted α,β-unsaturated ketones and the corresponding epoxide products were evaporated and purified by employing a silica gel column chromatography (ethyl acetate/n-hexane) to obtain the desired products.
GO has been synthesized using the modified Hummer’s method. Hummer’s method is an efficient and reliable method for producing GO through the oxidation of graphite powder with potassium permanganate in the presence of sodium nitrate in a solution of sulfuric acid47. Thereafter GO has been transformed into N-GOQDs by a “top-down” oxidative fragmentation route in the presence of aqueous H2O2 and N-(aminomethyl)methanediamine (Supplementary Fig. S1). Finally, N-GOQDs/OH− has been prepared by converting the amine groups to quaternary methyl ammonium iodide followed by ion-exchange with aqueous KOH solution, as shown in Fig. 2. The presence of various functional groups on the surface of the N-GOQDs/OH− imparts high solubility in water and polar organic solvents, therefore, it can act as a pseudo-homogeneous catalyst.
The as-synthesized GOQDs was characterized using Fourier transform infrared spectroscopy (FTIR), Energy-dispersive X-ray spectroscopy (EDX), Transmission electron microscopy (TEM), Scanning electron microscopy (SEM), X-ray photoelectron spectroscopy (XPS) and Fluorescence spectroscopy.
Figure 1a shows FT-IR spectra of the prepared GO and N-GOQDs/OH−. GO shows its characteristic peaks at 3375, 1731, 1618, and 1044 cm−1 arising due to O–H, C=O, C=C and C–O–C of the epoxides, respectively. After the oxidative fragmentation, the carbonyl and epoxide peaks disappear (1731 and 1044 cm−1 respectively)45. The presence of –OH and –NH group in N-GOQDs/OH− was confirmed by the appearance of broad peak centered between 3000 cm−1 and 3500 cm−1. The strong signal at 1668 cm−1 can be correspond to the stretching vibration of C=N and C=O bonds, and the peak at 1384 cm−1 arise due to stretching vibration of C and N bond48. The presence of aliphatic C–H group is confirmed by the peak at 2927 cm−1. Therefore, the FT-IR image indicates that the compounds that contain nitrogen atoms are modified on the surface of the GOQDs. In the 1H NMR spectrum, dispersed in D2O, the presence of quaternary ammonium hydroxide groups could be identified (Fig. 1b).
(a) FT-IR spectra of the GO and N-GOQDs/OH− (b) 1H NMR spectrum of the N-GOQDs/OH−.
Figure 2a,b shows TEM images of the obtained GO and the N-GOQDs/OH− and Fig. 2c shows the particle size distribution. The average the N-GOQDs/OH− size must be significantly smaller than the GO. The TEM images (Fig. 2b) clearly reveal the N-GOQDs/OH− are nearly spherical in shape and mono-dispersed with particle sizes smaller than 10 nm whose mean size is very smaller than GO (Fig. 2a). Figures 2d,e show SEM images of the prepared GO and N-GOQDs/OH−, respectively. According to the FE-SEM image (Fig. 2e) of the GOQDs show sphere-shaped morphology.
The TEM images of (a) the obtained GO, (b) N-GOQDs/OH−, and (c) particle size distribution, and FE-SEM image of (d) the obtained GO, (e) N-GOQDs/OH−.
To study the distribution and chemical composition of the N-GOQDs/OH−, EDX analysis was performed (Supplementary Fig. S2). A SEM image of the same region as the EDX is shown in Supplementary Fig. S2a. The elemental peaks attributed to carbon, oxygen, and nitrogen in the EDX spectrum confirmed the presence of these elements in the obtained N-GOQDs/OH− (Supplementary Fig. S2b). Additionally, the EDX spectrum reveals the presence of an ignorable amount of potassium and calcium impurities (0.05 and 0.10 atom percent, respectively) (Supplementary Table S1). The presence of potassium can be related to an extremely small amount of KOH not removed in the dialysis step and the presence of calcium is a sign of environmental impurity in the catalyst identification stage. Therefore, the EDX spectrum confirmed good anchoring and stability of compounds that contain nitrogen atoms on GOQDs. Additionally, it can be inferred from the mapping images that elements of carbon, oxygen, and nitrogen are widespread in the area of N-GOQDs/OH− because of the uniform formation of N-GOQDs/OH− (Supplementary Fig. S2c).
Fluorescence spectroscopy is a simple and reliable methodology to confirm the quantum confined properties of semiconductor QDs45,49. The fluorescence response of the N-GOQDs/OH− with regard to a variety of excitation wavelengths between 320 and 540 nm is displayed in Fig. 3. Consistent with previous research, the fluorescence intensity of the N-GOQDs/OH− sample first increased and then decreased (Fig. 3). The samples displayed exhibited the strongest fluorescence peak with maximum emission centered at 445 nm at an excitation wavelength of 360 nm. The results clearly indicated the successful synthesis of the N-GOQDs/OH− samples. Furthermore, photographs of an aqueous dispersion of N-GOQDs/OH− under room light (left) and under 365 nm UV irradiation (right) shown in the figure.
The fluorescence spectrum of the N-GOQDs/OH−.
The atomic chemical states of synthesized N-GOQDs/OH− sample was studied by XPS (Fig. 4). The full XPS spectra of N-GOQDs/OH− clearly reveals the existence of carbon, oxygen and nitrogen (Supplementary Fig. S3). C1s spectrum shows peaks at BEs of 284 eV (referring to C–C and C=C), 286 eV (referring to C–O and C–N) and 288 eV (referring to C=O) (Fig. 4). The analysis of O1s shows peaks at BEs of 530 eV (assigned to C–O and C=O), and 532 eV (assigned to O–H) (Fig. 4). The N1s shows peaks at 400 eV and 402 eV corresponding to C–N and the quaternary ammonium group, respectively (Fig. 4)15,45,50.
High resolution XPS spectra of C1s, O1s, N1s.
The catalytic activity of N-GOQDs/OH−, as a pseudo-homogeneous catalyst, was evaluated in epoxidation of α,β-unsaturated ketones. Initially, the epoxidation of benzalacetophenone was studied as standard substrate with the prepared nanocatalyst (Table 1). At the outset, many experimental parameters, such as catalyst amount, the H2O2/α,β-unsaturated ketones molar ratios and solvent have been studied in order to determine the optimal experimental conditions. The effect of reaction conditions on epoxidation of benzalacetophenone (1 mmol) with N-GOQDs/OH− summarized in Table 1. As can be seen from the results in Table 1, in the absence of the catalyst, and in the presence of GO or N-GOQDs, no product obtained (Table 1, Entries 1–3) and an increase in the amount of the catalyst improve the yields significantly (Table 2, Entries 4–6). The effect of addition of H2O2 amount on the reaction was also studied. The yield significant improvements of epoxide product were observed with increasing amount of H2O2 (Entries 6–10). Investigating the role of solvent show that the protic solvents reduces the epoxide products yields (Entries 11–13). After careful examinations, the best result was obtained by using 4 mL of aqueous solution of catalyst, and in the presence of H2O2 (3 mmol) in 1,4-dioxane at ambient temperature (Table 1, Entry 6).
Under the optimized conditions, in a simple experimental procedure the transformations of a series of α,β-unsaturated ketones into the corresponding epoxides occurred in almost high yields. The results are summarized in Table 2. The present procedure is general as a wide range of α,β-unsaturated ketones derivatives with electron-donating and electron-withdrawing groups reacted easily with H2O2 at room temperature to afford the corresponding α,β-epoxy ketones in good to high yields and electronic effect of the substituents was not observed.
According to the literature, plausible mechanisms for the epoxidation of α,β-unsaturated ketones is proposed as shown in Fig. 5. The OH− plays an essential role through formed HOO− specie. On the other hand, the N-GOQDs/OH− activate the α,β-unsaturated ketones through hydrogen-bond interaction. The reaction is followed by the nucleophile attack of the HOO− and form a hydroperoxide enolate. Finally, the reaction is completed by lost the OH- and formed the epoxide ring53,54,55,56.
The plausible mechanism for the epoxidation reaction.
After completion of the reaction in a homogeneous system, the reaction mixture was diluted with water, and the product was extracted with ethyl acetate. Since the catalyst is completely soluble in water, the catalyst was retained in the aqueous phase. The extracted catalyst in the aqueous layer was concentrated under vacuum to a final volume of 4 mL to reuse in another run. Recycling experiments confirmed the acceptable reusability and chemical stability of the catalyst since the recovered catalyst can be used during four runs (Fig. 6).
Reusability of N-GOQDs/OH− for the epoxidation of benzalacetophenone.
Table 3 lists the comparison of catalytic performance of N-GOQDs/OH− as a pseudo-homogeneous catalyst for the epoxidation of benzalacetophenone with various reported catalysts. Compared with some data in the literature, the N-GOQDs/OH− revealed appropriate catalytic performance and good reusability for the epoxidation of benzalacetophenone.
In summary, quaternary ammonium hydroxide‐functionalized GOQDs has been prepared. The provided GOQDs were characterized using 1H NMR, FT-IR, TEM, SEM, XPS, EDX mapping, and fluorescence spectroscopy. On the whole, results of these analysis support the expected structure of N-GOQDs/OH−. The catalyst was found to be active towards epoxidation of α,β-unsaturated ketones in the presence of aqueous H2O2 as a green oxidant, at ambient temperature. The corresponding epoxide products were obtained in good to high yields. Due to the presence of various functional groups on the surface of the N-GOQDs/OH− imparts high solubility in water and polar organic solvents. Therefore substrates and the catalyst can create a homogeneous phase, this helps to achieve a high efficiency of the catalyst. This pseudo-homogeneous catalyst will be widely expected to use for future catalytic applications in oxidation reactions of organic compounds.
All data generated or analyzed during this study are included in this published article [and its supplementary information files].
Varma, R. S. Greener and sustainable trends in synthesis of organics and nanomaterials. ACS Sustain. Chem. Eng. 4, 5866–5878 (2016).
Article CAS PubMed PubMed Central Google Scholar
Chng, L. L., Erathodiyil, N. & Ying, J. Y. Nanostructured catalysts for organic transformations. Acc. Chem. Res. 46, 1825–1837 (2013).
Article CAS PubMed Google Scholar
Garcia, H. Allotropic carbon nanoforms as advanced metal-free catalysts or as supports. Adv. Chem. 2014, 906781 (2014).
Du, J. T. et al. ZnO nanodispersion as pseudohomogeneous catalyst for alcoholysis of polyethylene terephthalate. Chem. Eng. Sci. 220, 115642 (2020).
Karimi, B. & Ramezanzadeh, B. A comparative study on the effects of ultrathin luminescent graphene oxide quantum dot (GOQD) and graphene oxide (GO) nanosheets on the interfacial interactions and mechanical properties of an epoxy composite. J. Colloid Interface Sci. 493, 62–76 (2017).
Article ADS CAS PubMed Google Scholar
Chen, W. et al. Synthesis and applications of graphene quantum dots: A review. Nanotechnol. Rev. 7, 157–185 (2018).
Shen, J., Zhu, Y., Yang, X. & Li, C. Graphene quantum dots: Emergent nanolights for bioimaging, sensors, catalysis and photovoltaic devices. ChemComm. 48, 3686–3699 (2012).
Xie, R. et al. Graphene quantum dots as smart probes for biosensing. Anal. Methods 8, 4001–4016 (2016).
Cheng, T. Y., Chou, F. P., Huang, S. C., Chang, C. Y. & Wu, T. K. Electroluminescence and photocatalytic hydrogen evolution of S, N co-doped graphene oxide quantum dots. J. Mater. Chem. 10, 3650–3658 (2022).
Sun, Y. et al. Large scale preparation of graphene quantum dots from graphite with tunable fluorescence properties. Phys. Chem. Chem. Phys. 15, 9907–9913 (2013).
Article CAS PubMed Google Scholar
Pan, D. et al. Cutting sp 2 clusters in graphene sheets into colloidal graphene quantum dots with strong green fluorescence. J. Mater. Chem. 22, 3314–3318 (2012).
Liu, Y. et al. Gram-scale synthesis of graphene quantum dots from single carbon atoms growth via energetic material deflagration. Chem. Mater. 27, 4319–4327 (2015).
Farshbaf, M. et al. Carbon quantum dots: Recent progresses on synthesis, surface modification and applications. Artif. Cells Nanomed. Biotechnol. 46, 1331–1348 (2018).
Article CAS PubMed Google Scholar
Hadian-Dehkordi, L. et al. Amphiphilic carbon quantum dots as a bridge to a pseudohomogeneous catalyst for selective oxidative cracking of alkenes to aldehydes: A nonmetallic oxidation system. ACS Appl. Mater. Interfaces 12, 31360–31371 (2020).
Article CAS PubMed Google Scholar
Rezaei, A. et al. Pseudohomogeneous metallic catalyst based on tungstate-decorated amphiphilic carbon quantum dots for selective oxidative scission of alkenes to aldehyde. Sci. Rep. 11, 4411 (2021).
Article ADS CAS PubMed PubMed Central Google Scholar
Mohammadi, M., Khazaei, A., Rezaei, A., Huajun, Z. & Xuwei, S. Ionic-liquid-modified carbon quantum dots as a support for the immobilization of tungstate ions (WO42–): Heterogeneous nanocatalysts for the oxidation of alcohols in water. ACS Sustain. Chem. Eng. 7, 5283–5291 (2019).
Mohammadi , M. , Rezaei , A. , Khazaei , A. , Xuwei , S. & Huajun , Z. Targeted development of sustainable green catalysts for oxidation of alcohols via tungstate-decorated multifunctional amphiphilic carbon quantum dots .ACS Appl.Mater.Interfaces 11, 33194–33206 (2019).
Article CAS PubMed Google Scholar
Bayan, R. & Karak, N. Photo-assisted synthesis of a Pd–Ag@CQD nanohybrid and its catalytic efficiency in promoting the suzuki–miyaura cross-coupling reaction under ligand-free and ambient conditions. ACS Omega 2, 8868–8876 (2017).
Article CAS PubMed PubMed Central Google Scholar
Zhang, M. et al. Peroxide- and transition metal-free electrochemical synthesis of α,β-epoxy ketones. Org. Biomol. Chem. 19, 2481–2486 (2021).
Article CAS PubMed Google Scholar
Mirzaei, H. & Emami, S. Recent advances of cytotoxic chalconoids targeting tubulin polymerization: Synthesis and biological activity. Eur. J. Med. Chem. 121, 610–639 (2016).
Article CAS PubMed Google Scholar
Nettles, J. H. et al. The binding mode of epothilone A on α,ß-tubulin by electron crystallography. Science 305, 866–869 (2004).
Article ADS CAS PubMed Google Scholar
Hayashi, K. I., Nakagawa, M. & Nakayama, M. Nisamycin, a new manumycin group antibiotic from Streptomyces sp. K106. I. Taxonomy, fermentation, isolation, physico-chemical and biological propertises. J. Antibiot. 47, 1104–1109 (1994).
Hanada, K. et al. Isolation and characterization of E–64, a new thiol protease inhibitor. Agric. Biol. Chem. 42, 523–528 (1987).
Bauer, K., Garbe, D., Surburg, H. Common Fragrance and Flavor Materials: Preparation, Properties and Uses (Wiley, 2008).
Sienel, G., Rieth, R. & Rowbottom, K. T. Ullmann’s Encyclopedia of Organic Chemicals (Wiley-VCH, 1999).
Kim, D. Y. et al. Enantioselective epoxidation of α,β-unsaturated ketones by phase-transfer catalysis using chiral quaternary ammonium salts. Synth. Commun. 33, 435–443 (2003).
Reddy, V. K., Haritha, B. & Yamashita, M. Highly diastereoselective epoxidation of α,β-unsaturated carbonyl compounds using sodium peroxide. Lett. Org. Chem. 2, 128–131 (2005).
Andrus, M. B. & Poehlein, B. W. Epoxidation of olefins with peracid at low temperature with copper catalysis. Tetrahedron Lett. 41, 1013–1014 (2000).
Geng, X. L., Wang, Z., Li, X. Q. & Zhang, C. A simple method for epoxidation of olefins using sodium chlorite as an oxidant without a catalyst. J. Org. Chem. 70, 9610–9613 (2005).
Article CAS PubMed Google Scholar
Allen, J. V., Drauz, K. H., Flood, R. W., Roberts, S. M. & Skidmore, J. Polyamino acid-catalysed asymmetric epoxidation: Sodium percarbonate as a source of base and oxidant. Tetrahedron Lett. 40, 5417–5420 (1999).
Hashimoto, N. & Kanda, A. Practical and environmentally friendly epoxidation of olefins using oxone. Org. Process Res. Dev. 6, 405–406 (2002).
Jakka, K., Liu, J. & Zhao, C. G. Facile epoxidation of α,β-unsaturated ketones with cyclohexylidenebishydroperoxide. Tetrahedron Lett. 48, 1395–1398 (2007).
Article CAS PubMed PubMed Central Google Scholar
Ye, J., Wang, Y., Chen, J. & Liang, X. Trichloroisocyanuric acid: A convenient oxidation reagent for phase-transfer catalytic epoxidation of enones under non-aqueous conditions. Adv. Synth. Catal. 346, 691–696 (2004).
Baumstark, A. L. & Harden, D. B. Jr. Epoxidation of α,β-unsaturated carbonyl compounds by dimethyldioxirane. J. Org. Chem. 58, 7615–7618 (1993).
Targhan, H., Evans, P. & Bahrami, K. A review of the role of hydrogen peroxide in organic transformations. J. Ind. Eng. Chem. 104, 295–332 (2021).
Jin, H. et al. Efficient epoxidation of chalcones with urea-hydrogen peroxide under ultrasound irradiation. Ultrason. Sonochem. 16, 304–307 (2009).
Article CAS PubMed Google Scholar
Wang, B., Kang, Y. R., Yang, L. M. & Suo, J. S. Epoxidation of α,β-unsaturated carbonyl compounds in ionic liquid/water biphasic system under mild conditions. J. Mol. Catal. A Chem. 203, 29–36 (2003).
Article ADS CAS Google Scholar
Rinaldi, R., de Oliveira, H. F., Schumann, H. & Schuchardt, U. Homogeneously catalyzed epoxidation of α,β-unsaturated ketones using simple aluminum salts and aqueous H2O2—Is it possible. J. Mol. Catal. A Chem. 307, 1–8 (2009).
Gao, Q., Ding, Y., Liu, H. & Suo, J. Epoxidation of electron-deficient α,β-unsaturated carbonyl compounds over Keggin heteropoly compounds with aqueous H2O2. J. Chem. Res. 11, 716–718 (2005).
Yang, S. G., Hwang, J. P., Park, M. Y., Lee, K. & Kim, Y. H. Highly efficient epoxidation of electron-deficient olefins with tetrabutylammonium peroxydisulfate. Tetrahedron 63, 5184–5188 (2007).
Karthikeyan, P. et al. A novel l -asparaginyl Amido ethyl methyl imidazolium bromide catalyst for heterogeneous epoxidation of α,β-unsaturated ketones. J. Mol. Liq. 172, 136–139 (2012).
Bérubé, C., Barbeau, X., Lagüe, P. & Voyer, N. Revisiting the Juliá–Colonna enantioselective epoxidation: supramolecular catalysis in water.Chem.Common.53, 5099–5102 (2017).
Wang, X., Reisinger, C. M. & List, B. Catalytic asymmetric epoxidation of cyclic enones. J. Am. Chem. Soc. 130, 6070–6071 (2008).
Article CAS PubMed Google Scholar
Bahrami, K. & Targhan, H. A new strategy to design a graphene oxide supported palladium complex as a new heterogeneous nanocatalyst and application in carbon–carbon and carbon-heteroatom cross-coupling reactions. Appl. Organomet. Chem. 33, e4842 (2019).
El-Hnayn, R. et al. One-step synthesis of diamine-functionalized graphene quantum dots from graphene oxide and their chelating and antioxidant activities. Nanomaterials 10, 104 (2020).
Article CAS PubMed PubMed Central Google Scholar
Muhammad, I., Mannathan, S. & Sasidharan, M. Quaternary ammonium hydroxide-functionalized g-C3N4 catalyst for aerobic hydroxylation of arylboronic acids to phenols. J. Chin. Chem. Soc. 67, 1470–1476 (2020).
Sahudin , MR , Makhsin , SR , Ayub , MA , Saad , NH , Rani , PA , Zourob , M. In 2021 IEEE Regional Symposium on Micro and Nanoelectronics (RSM) (2021).
Shen, T.-Y., Jia, P.-Y., Chen, D.-S. & Wang, L.-N. Hydrothermal synthesis of N-doped carbon quantum dots and their application in ion-detection and cell-imaging. Spectrochim. Acta Part A Mol. Biomol. Spectrosc. 248, 119282 (2021).
Mutavdžić, D. et al. Determination of the size of quantum dots by fluorescence spectroscopy. Analyst 136, 2391–2396 (2011).
Article ADS PubMed Google Scholar
Li, G. et al. Ultrathin composite membrane of alkaline polymer electrolyte for fuel cell applications. J. Mater. Chem. A. 1, 12497–12502 (2013).
Lakouraj, M. M., Movassagh, B. & Bahrami, K. Mild and convenient epoxidation of α,β-unsaturated ketones by amberlyst A-26 supported hydroperoxide. Synth. Commun. 31, 1237–1242 (2001).
Adam, W., Hadjiarapoglou, L. & Smerz, A. Dioxirane epoxidation of α,β-unsaturated ketones. Chem. Ber. 124, 227–232 (1991).
Lu, Y., Zheng, C., Yang, Y., Zhao, G. & Zou, G. Highly enantioselective epoxidation of α,β-unsaturated ketones catalyzed by primary-secondary diamines. Adv. Synth. Catal. 353, 3129–3133 (2011).
Kelly, D. R. & Roberts, S. M. The mechanism of polyleucine catalysed asymmetric epoxidation. Chem. Commun. 18, 2018–2020 (2004).
Rajput, J. K. & Kaur, G. Silicotungstic acid catalysed Claisen Schmidt condensation reaction: An efficient protocol for synthesis of 1,3-diaryl-2-propenones. Tetrahedron Lett. 53, 646–649 (2012).
Banfi, S., Colonna, S., Molinari, H., Julia, S. & Guixer, J. Asymmetric epoxidation of electron-poor olefins-V: Influence on stereoselectivity of the structure of poly-α-aminoacids used as catalysts. Tetrahedron 40, 5207–5211 (1984).
Yamaguchi , K. , Mori , K. , Mizugaki , T. , Ebitani , K. & Kaneda , K. Epoxidation of α,β-unsaturated ketones using hydrogen peroxide in the presence of basic hydrotalcite catalysts.J. Org.Chem.Rev. 65, 6897–6903 (2000).
Article CAS PubMed Google Scholar
Pourali, A. R. Polymer-supported oxone and tert-butyl hydroperoxide: New reagents for the epoxidation of α,β-unsaturated aldehydes and ketones. Mendeleev Commun. 20, 113–115 (2010).
Wu, Y. et al. Visible light-induced aerobic epoxidation of α,β-unsaturated ketones mediated by amidines. J. Org. Chem. 83, 13051–13062 (2018).
Article CAS PubMed Google Scholar
The authors acknowledge the Razi University Research Council for support of this work.
Nanoscience and Nanotechnology Research Center (NNRC), Razi University, Kermanshah, 67144-14971, Iran
Mohammed Salim Mohammed & Kiumars Bahrami
Department of Organic Chemistry, Faculty of Chemistry, Razi University, Kermanshah, 67144-14971, Iran
Homa Targhan & Kiumars Bahrami
You can also search for this author in PubMed Google Scholar
You can also search for this author in PubMed Google Scholar
You can also search for this author in PubMed Google Scholar
K.B. conceived the original idea and supervised the project. M.S.M. carried out the experiments. M.S.M. and H.T. wrote the main manuscript text. All authors reviewed the manuscript.
The authors declare no competing interests.
Springer Nature remains neutral with regard to jurisdictional claims in published maps and institutional affiliations.
Open Access This article is licensed under a Creative Commons Attribution 4.0 International License, which permits use, sharing, adaptation, distribution and reproduction in any medium or format, as long as you give appropriate credit to the original author(s) and the source, provide a link to the Creative Commons licence, and indicate if changes were made. The images or other third party material in this article are included in the article's Creative Commons licence, unless indicated otherwise in a credit line to the material. If material is not included in the article's Creative Commons licence and your intended use is not permitted by statutory regulation or exceeds the permitted use, you will need to obtain permission directly from the copyright holder. To view a copy of this licence, visit http://creativecommons.org/licenses/by/4.0/.
Mohammed, M.S., Targhan, H. & Bahrami, K. Design and introduction of quaternary ammonium hydroxide‐functionalized graphene oxide quantum dots as a pseudo-homogeneous catalyst for epoxidation of α,β-unsaturated ketones. Sci Rep 13, 8140 (2023). https://doi.org/10.1038/s41598-023-34635-5
DOI: https://doi.org/10.1038/s41598-023-34635-5
Anyone you share the following link with will be able to read this content:
Sorry, a shareable link is not currently available for this article.
Provided by the Springer Nature SharedIt content-sharing initiative
By submitting a comment you agree to abide by our Terms and Community Guidelines. If you find something abusive or that does not comply with our terms or guidelines please flag it as inappropriate.
Scientific Reports (Sci Rep) ISSN 2045-2322 (online)

Dmtcl 44' Dimethoxytrityl Sign up for the Nature Briefing newsletter — what matters in science, free to your inbox daily.